ABRUPT
CHANGES IN THE EARTH’S CLIMATE SYSTEM
ABRUPT CHANGE IN SEA LEVEL
Abrupt change in sea
level
Population densities in coastal regions and on islands are
about three times higher than the global average, with approximately 23% of the
world’s population living within 100 kilometers (km) distance of the coast and
>100 meters (m) above sea level (Nicholls et al., 2007). This allows even small sea level rise to have
significant societal and economic impacts through coastal erosion, increased
susceptibility to storm surges and resulting flooding, ground-water
contamination by salt intrusion, loss of coastal wetlands, and other issues
(fig. 1.2).
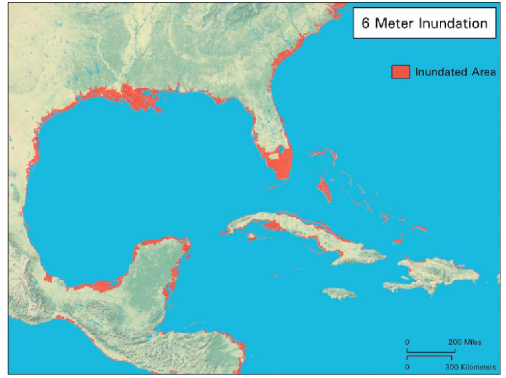
Figure 1.2. Portions (shown in red) of the Southeastern United States, Central America, and the
Caribbean surrounding the Gulf of Mexico that would be inundated by a 6-mete sea level rise
(from Rowley et al., 2007). Note that additional changes in the position of the coastline may occur in
response to erosion from the rising sea level.
An increase in global sea level largely reflects a
contribution from water expansion from warming, and from the melting of land
ice which dominates the actual addition of water to the oceans. Over the last century, the global average sea
rose at a rate of ~1.7 ± 0.5 millimeters per year (mm yrˉ1). However, the rate of global sea level rise
for the period 1993 to 2003 accelerated to 3.1 ±0.7 mm yrˉ1, reflecting
either variability on decadal time scales or an increase in the longer term
trend. Relative to the period 1961-2003,
estimates of the contributions from thermal expansion and from glaciers and ice
sheets indicate that increases in both of these sources contributed to the
acceleration in global sea level rise that characterized the 1992-2003 period
(Bindoff et al., 2007).
By the end of the 21st century and in the absence
of ice-dynamical contribution, the IPCC AR4 projects sea level to rise by 0.28
± 0.10 m to 0.42 ± 0.16 m in response to additional global warming, with the
contribution from thermal expansion accounting for 70-75% of this rise (Meehl
et al., 2007). Projections for
contributions from ice sheets are based on models that emphasize accumulation
and surface melting in controlling the amount of mass gained and lost by ice
sheets (mass balance), with different relative contributions for the Greenland and Antarctic ice sheets. Because the increase in mass loss (ablation)
is greater than the increase in mass gain (accumulation), the Greenland Ice
Sheet is projected to contribute to a positive sea level rise and may melt
entirely from future global warming (Ridley et al., 2005). In contract, the Antarctic Ice Sheet is
projected to grow through increased accumulation relative to ablation and thus
contribute to a negative sea level rise.
The net projected effect on global sea level from these two differing
ice-sheet responses to global warming over the remainder of this century is to
nearly cancel each other out.
Accordingly, the primary contribution to sea level rise from projected
mass changes in the IPCC AR4 is associated with retreat of glaciers and ice
caps (Meehl et al., 2007).
Rahmstorf (2007) used the relation between 20th
century sea level rise and global mean surface temperature increase to predict
a sea level rise of 0.5 to 1.4 m above the 1990 level by the end of the 21st
century, considerably higher than the projections by the IPCC AR4 (Meehl et
al., 2007). Insofar as the contribution to 20th century sea
level rise from melting land ice is thought to have been dominated by glaciers
and ice caps (Bindoff et al., 2007), the Rahmstorf (2007) projection
does not include the possible contribution to sea level rise from ice sheets.
Recent observations of startling changes at the margins of
the Greenland and Antarctic ice sheets
indicate that dynamic responses to warming may play a much greater role in the
future mass balance of ice sheets than considered in current numerical
projections of sea level rise. Ice-sheet
models used as the basis for the IPCC AR4 numerical projections did not include
the physical processes that may be governing these dynamical responses, but if
they prove to be significant to the long-term mass balance of the ice sheets,
sea level projections will likely need to be revised upwards
substantially. By implicitly excluding
the potential contribution from ice sheets, the Rahmstor (2007) estimate will
also likely need to be revised upwards if dynamical processes cause future
ice-sheet mass balance to become more negative.
The Greenland Ice Sheet is losing mass and very likely on an
accelerated path since the mid-1990s.
Observations show that Greenland is thickening
at high elevations, because of an increase in snowfall, but that this gain is
more than offset by an accelerating mass loss at the coastal margins, with a large
component from rapidly thinning and accelerating outlet glaciers. The
mass balance of the Greenland Ice Sheet during the period with good
observations indicates that the loss increased from 100 gigatons per year (Gt
a–1) (where 360 Gt of ice = 1 mm of sea level) in the late 1990s to more than
200 Gt a–1 for the most recent observations in 2006.
Determination of the mass budget of the Antarctic ice sheet
is not as advanced as that for Greenland. The mass balance for Antarctica
as a whole has experienced a net loss of about 80 Gt a-1 in the mid-1990s,
increasing to almost 130 Gt a-1 in the mid-2000s. There is little surface melting in
Antarctica, but substantial ice losses are occurring from West Antarctica and
the Antarctic Peninsula primarily in response to changing ice dynamics.
The record of past changes provides important insight to the
behavior of large ice sheets during warming.
At the last glacial maximum about 21,000 years ago, ice volume and area
were about 2.5 times modern.
Deglaciation was forced by warming from changes in the Earth’s orbital
parameters, increasing greenhouse gas concentrations, and attendant
feedbacks. Deglacial sea level rise
averaged 10mm a-1, but with variations including two extraordinary
episodes at 19,000 years ago (ka) and 14.5 ka when peak rates potentially
exceeded 50mm a-1 (Fairbanks, 1989; Yokoyama et al., 2000). Each of these "meltwater pulses” added the
equivalent of 1.5 to 3 Greenland ice sheets (~10-20 m) to the oceans over a
one- to five- century period, clearly demonstrating the potential for ice
sheets to cause rapid and large sea level changes.
The primary factor that raises concerns about the potential
of future abrupt changes in sea level is that large areas of modern
ice sheets are currently grounded below sea level. Where it exists, it is this
condition that lends itself to many of the processes that can lead to rapid ice-sheet
changes, especially with regard to atmosphere-ocean-ice interactions that may affect
ice shelves and calving fronts of glaciers terminating in water (tidewater glaciers).
An important aspect of these marine-based ice sheets is that the beds of ice
sheets grounded below sea level tend to deepen inland. The grounding line is
the critical juncture that separates ice that is thick enough to remain grounded
from either an ice shelf or a calving front. In the absence of stabilizing
factors, this configuration indicates that marine ice sheets are inherently
unstable, whereby small changes in climate could trigger irreversible retreat
of the grounding line.
The amount of retreat clearly depends on how far inland
glaciers remain below sea level. Of
greatest concern is the West Antarctic Ice Sheet, with 5 to 6m sea level
equivalent, where much of the base of the ice sheet is grounded well below sea
level, with deeper trenches lying well inland of their grounding lines. A similar situation applies to the entire
Wilkes Land sector of East Antarctica. In Greenland
a number of outlet glaciers remain below sea level, indicating that glacier
retreat by this process will continue for some time. A notable example is Greenland’s largest
outlet glacier, Jakobshavn Isbrae, which appears to tap into the central region
of Greenland that is below sea level. Accelerated ice discharge is possible through
such outlet glaciers, but we consider the potential for destabilization of the
Greenland Ice sheet by this mechanism to be very unlikely.
The key requirement for stabilizing grounding lines of
marine-based ice sheets appears to be the presence of an extension of floating
ice beyond the grounding line, referred to as an ice shelf. A thinning
ice shelf results in ice-sheet ungrounding, which is the main cause
of the ice acceleration because it has a large effect on the force
balance near the ice front. Recent rapid hanges in
marginal regions of both ice sheets are characterized mainly by
acceleration and thinning, with some glacier
velocities increasing more than twofold. Many of these
glacier accelerations
closely followed reduction or loss of ice shelves. If glacier
acceleration caused by thinning ice shelves can be sustained
over many centuries, sea level will rise more rapidly than currently
estimated.
Such behavior was predicted almost 30 years ago
by Mercer (1978) but was discounted as recently as the IPCC Third Assessment
Report (Church
et al., 2001) by most of the glaciological community based
largely on results from prevailin model simulations. Considerable
effort is
now underway to improve the models, but it is far from
complete, leaving us unable to make reliable predictions of ice-sheet
responses to a warming climate if such glacier
accelerations were to increase in size and
frequency.
A nonlinear response of ice-shelf melting to increasing
ocean temperatures is a central tenet in the scenario for abrupt sea-level rise
arising from ocean-ice-shelf interactions.
Significant changes in ice-shelf thickness are most readily caused by
changes in basal melting. The
susceptibility of ice shelves to high melt rates and to collapse is a function
of the presence of warm waters entering the cavities beneath ice shelves. Future changes in ocean circulation and ocean
temperatures will produce changes in basal melting, but the magnitude of these
changes is currently neither modeled nor predicated.
Another mechanism that can potentially increase the
sensitivity of ice sheets to climate change involves enhanced flow of the
ice over its bed due to the presence of pressurized water, a process
known as sliding. Where such basal flow is enabled, total ice flow
rates may increase by 1 to 10 orders of magnitude, significantly decreasing
the response time of an ice sheet to a climate or ice-marginal
perturbation.
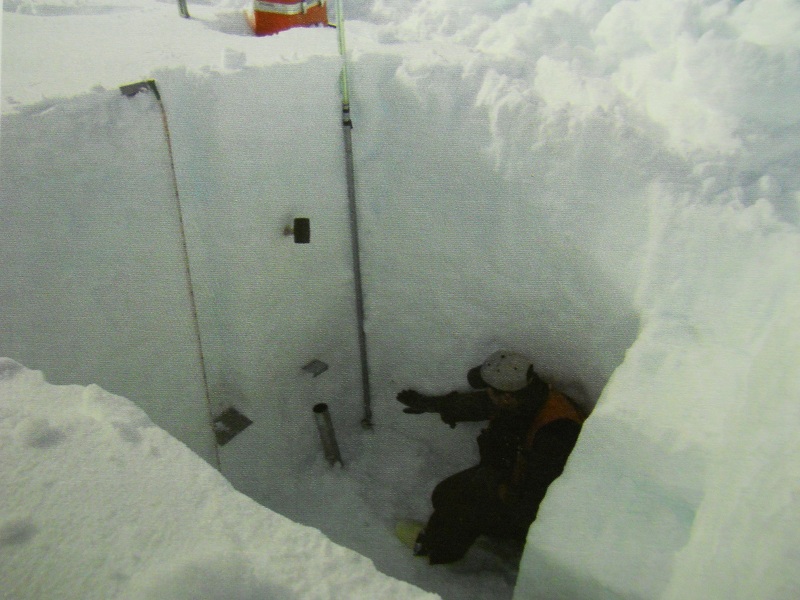
Recent data from Greenland
show a high correlation between periods of heavy surface
melting and
an increase in glacier velocity (Zwally et al., 2002).
A possible cause for this relation is rapid drainage of
surface meltwater to the glacier bed, where it enhances lubrication
and basal sliding. There has been a significant increase in meltwater
runoff from the Greenland Ice Sheet for the 1998–2007 period
compared to the previous three decades (Fig.
1.3). Total melt area is continuing to increase during
the melt season and has already reached up to 50% of the Greenland Ice
Sheet; further increase in Arctic temperatures will very likely
continue this process and will add additional runoff. Because water represents such an important control
on glacier flow, an increase in meltwater production in a
warmer climate will likely have major consequences on flow rate and
mass loss. Because sites of global deep
water formation occur immediately adjacent to the Greenland and Antarctic ice sheets, any
significant increase in freshwater fluxes from these ice
sheets may
induce changes in ocean heat transport and thus climate. This topic is
addressed in Chapter 4 of this report.
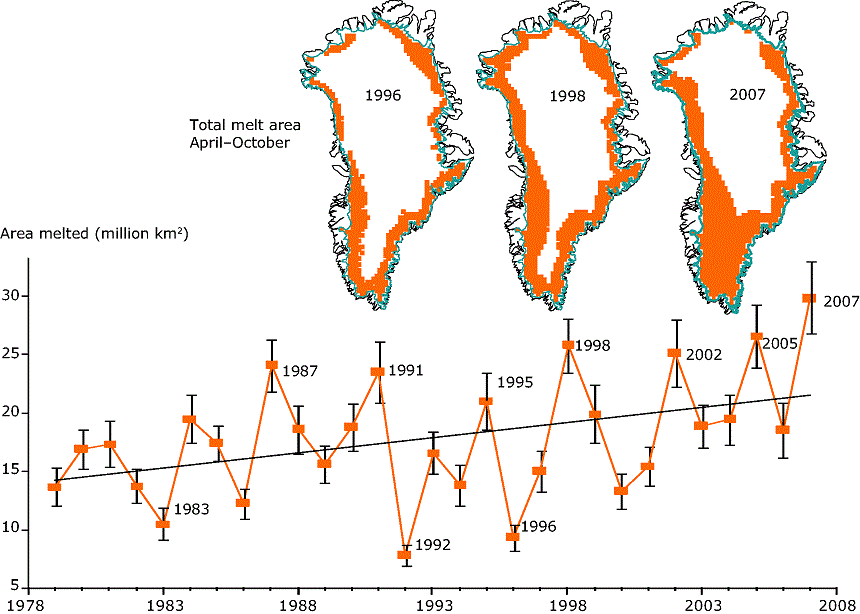
Figure 1.3. The graph shows the total melt area 1979 to 2007 for the Greenland ice sheet derived from passive
microwave satellite data. Error bars represent the 95% confidence interval. The map inserts display the area of melt
for 1996, 1998, and the record year 2007 (from K. Steffen, CIRES, University of Colorado).
SUMMARY
The Greenland and Antarctic
Ice Sheets are losing mass, likely at an accelerating rate. Much of the loss from Greenland is by
increased summer melting as temperatures rise, but an increasing proportion of
the combined mass loss is caused by increasing ice discharge from the ice-sheet
margins, indicating that dynamical responses to warming may play a much greater
role in the future mass balance of ice sheets than previously considered. The interaction of warm waters with the
periphery of the ice sheets is very likely one of the most significant
mechanisms to trigger an abrupt rise in global sea level. The potentially sensitive regions for rapid
changes in ice volume are thus likely those ice masses grounded below sea level
such as the West Antarctic Ice sheet or large glaciers in Greenland like the
Jakobshavn Isbrae with an over-deepened channel (channel below sea level, Fig.2.10) reaching far inland.
Ice-sheet models currently do not include the physical processes that
may be governing these dynamical responses, so quantitative assessment of their
possible contribution to sea level rise is not yet possible. If these processes prove to be significant to
the long-term mass balance of the ice sheets, however, current sea level
projections based on present-generation numerical models will likely need to be
revised substantially upwards.
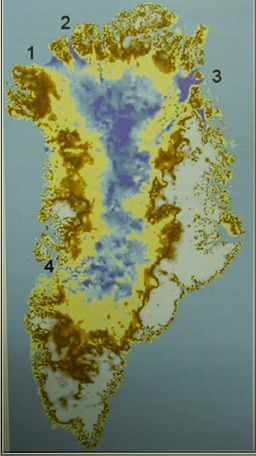
Figure 2.10. Bedrock topography for Greenland; areas
below sea level are shown in blue. Note the three channels
in the north (1: Humboldt Glacier; 2: Petermann Glacier;
3: 79-North Glacier or Nioghalvfjerdsfjorden Glacier) and
at the west coast (4: Jakobshavn Isbrae) connecting the
region below sea level with the ocean (Russell Huff and
Konrad Steffen, CIRES, University of Colorado at Boulder).
NOTE: The term "forcing”
is used throughout this report to indicate any mechanism that causes the
climate system to change, or respond. Examples of forcings discussed in this
report include freshwater forcing of ocean circulation and changes in
sea-surface temperatures and radiative forcing as a forcing of drought. As
defined by the IPCC Third Assessment Report (Church et al., 2001), radiative forcing refers to a change in the net radiation at
the top of the troposphere caused by a change in the solar radiation, the
infrared radiation, or other changes that affect the radiation energy absorbed
by the surface (e.g.,
changes in surface
reflection properties), resulting in a radiation imbalance. A positive
radiative forcing tends to warm the surface on average, whereas a negative radiative
forcing tends to cool it. Changes in GHG concentrations represent a radiative
forcing through their absorption and emission of infrared radiation.